Part 6: Redox Potential
This is a basic introduction to the redox potential in electrochemistry.
The topics are listed below:
- Redox Potential I: About HOMO and LUMO
- Redox Potential II: About Ferrocene
- Redox Potential III: Factors that determine redox potential
- Redox Potential IV: Organic transition metal complex
- Redox Potential V: Linear relationship between HOMO energy and redox potential
Redox Potential IV: Organic transition metal complex
Professor Noriyuki Watanabe
It is interesting to use organic transition metal complexes as materials to investigate the correspondence between redox potential and HOMO-LUMO.
Usually, the maximum number of valence electrons around a metal atom is 18 electrons (18-electron rule). Here, a six-coordinate, octahedral complex is considered. Due to the crystal field formed by the ligand, the 5 degenerate d orbitals of the central metal are split into the low-energy orbitals t2g (triple degenerate orbitals:dxy, dxz, dyz) and the high-energy eg orbitals (double degeneration orbitals:dz2 - Y2, dz2) (The energy difference between the two sets of degenerate orbits is the crystal field splitting energy Δ.). If the destabilizing electron pairing energy P is larger than the crystal field splitting energy Δ, in order to avoid electron pairing, d electrons will be sequentially filled into three t2g orbits and then into 2 eg orbits. Most of the electrons in d orbitals are in the state of parallel spins (high spin type). Conversely, if the crystal field splitting energy Δ is larger than the pairing energy P, even at the expense of the disadvantage of electron pairing energy, 6 electrons can enter into the more stable t2g orbits in paired distribution (low spin type).
When the redox center is a metal (HOMO and LUMO energy levels are from metal) atoms or ions, the redox potential will vary greatly depending on whether the HOMO or LUMO energy level is eg orbit or t2g orbit. If it is t2g orbit, the redox potential tends to be positive. Since the Crystal Field Theory originates from the electrostatic interaction formed by the charge of the ligand, it can only be considered as the interaction of σ-type molecular bonds.
Ligand Field Theory and Molecular Orbital Theory incorporating π-bond interaction are more realistic. Metals whose 3d, 4s, and 4p orbitals (in the case of the first transition metal) and the ligand orbitals (σ and π orbitals) have the same symmetry interact with each other. Generally, the d-orbital energy level of a metal is higher than the σ-orbital energy level of a ligand and lower than the empty π-orbital (semi-bonded orbital) energy level of a ligand.
When the π-type t2g orbital of a metal atom interacts with the π-type molecular empty orbital of a ligand molecule, the metal t2g orbital energy level is reduced. The difference between this orbital energy level and the orbital energy level of σ∗ (σ∗; anti-bonded molecular orbital, derived from eg orbitals of metal atoms) is called splitting energy Δ. It is known that the 18-electron rule is generally established from the valence orbital d5sp3 orbital of the metal participating in the bond (a total of 18 electrons because each 9 orbitals contains 2 electrons). When the π empty orbital energy level of the ligand (π∗; π anti-bond orbital of the ligand) is relatively low and close to the d orbital of the metal ion, the dπ (t2g) - π∗ (empty π) orbital interaction becomes larger. , the t2g orbital energy level is reduced, and the previously formed Crystal Field Splitting Energy Δ (split due to the interaction between such as 4s, 4p orbitals of the metal and the ligand σ-type orbitals) increases to Δ′ (Ligand Field Split Energy).
NH3 < NCR < PBu3 < PMe3 < P(OMe)3 < PPh3 < P(OPh)3 < PCl3 < PF3 < CNR < CO
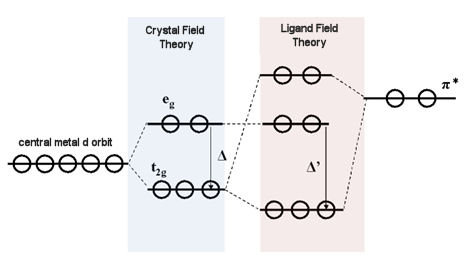
Fig.9-1. dπ (t2g) - π∗ interaction effect schematic diagram.
If the t2g orbital is at the HOM-LUMO level, the redox potential moves to positive due to its tending to a stable orientation (downward). For this reason, the redox potential of metal compounds coordinated with carbonyl or isocyanate is positive potential. On the other hand, the redox potential of amino compounds tends to show as a negative potential because the amino groups have low π acceptability and do not decrease the t2g orbitals energy level.~
As mentioned in previous issue, the reverse electron donor effect on the carbonyl group reduces the electron density around the metal, and the redox potential moves to positive, making it difficult to be oxidized, which can be explained by Ligand Field theory.